At issue is the effect of neutrinos,
which pervade every cubic inch of the
universe, on the rate at which the universe expands. Physicists also hope that
further neutrino discoveries will account for the Sun's "missing neutrinos,"
neutrinos predicted by theory but not
yet detected by observation.
A year ago at an international meeting at Takayama, Japan, a Japanese-American team of physicists disclosed
the first clear evidence that neutrinos
oscillate from one "flavor" to another
as they pass through Earth. The discovery, made with the help of a cathedral-size underground water tank called Super Kamiokande near the city of Kamioka, Japan, may shed light not only
on neutrinos but on the future expansion
rate and behavior of galaxies and the
universe.
In the past year physicists in North
America, Europe and Japan have been
racing each other to find independent
evidence confirming or refuting the
finding of neutrino oscillation, and several large laboratories have left the
starting gate in the quest.
The existence of neutrinos was predicted in 1930 and the particles were
actually discovered in 1956, but they
remain cloaked in enigmas. Physicists
have determined that in particle reactions, separate neutrino types are associated with three other types of lepton
particles: electrons, muons and tau particles. Thus, electron neutrinos (the kind
emitted by the Sun's nuclear reactions),
muon neutrinos (produced by laboratory accelerators) and tau electrons
(known by inference but never directly
detected) interact with matter in different ways.
Any experimental investigation of
neutrinos is extraordinarily difficult because these particles have no electric
charge and their mass, if not zero, must
be vanishingly small. This means that a
typical neutrino could pass through a
six-trillion-mile thickness of solid lead
with only a negligible chance of hitting
anything.
| 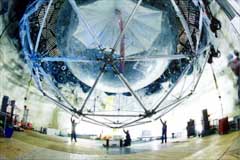
Sudbury Neutrino Observatory |
The vessel above holds heavy water at the the Sudbury Neutrino Observatory, 6,800 feet deep in a Canadian nickel mine.
|
Fortunately for scientists, however,
an occasional neutrino defies the astronomical odds and hits a bit of matter in
some detector -- a tank of water, an
atom of air or a huge block of Antarctic
ice, for example -- yielding a shower of
debris. Some of the debris leaves trails
of blue light as it streaks through
transparent objects or fluids. Measurements of this light, called Cherenkov radiation, allows scientists to
reconstruct the events that caused it.
Experiments under way promise
to shed light on the neutrino's apparent ability to transform itself into
different forms, a phenomenon that
could explain why the Sun's nuclear
furnace seems to emit many fewer
neutrinos than the process should
produce. It may simply be that the
solar neutrinos are reaching Earth
in the predicted numbers, but that
many of them have turned themselves into forms that are not revealed using conventional detectors.
On June 9 scientists announced
that the first neutrinos had been captured by a uniquely sensitive new
detector at the Sudbury Neutrino Observatory, known by the acronym
SNO, 6,800 feet below ground in the
Creighton nickel mine near Sudbury,
Ontario.
Like other large neutrino detectors, SNO mainly consists of a huge
water tank. What gives it greater
sensitivity for corralling neutrinos
than any similar detector is that in
place of ordinary water, it is filled
with 10,000 metric tons of heavy water, water in which each ordinary
hydrogen atom with a lone proton in
its nucleus is replaced by a hydrogen
isotope, called a deuteron, containing
a proton and a neutron.
Unlike ordinary water, which can
snag only one "flavor," or type of
neutrino, heavy water is sensitive to
all three neutrino flavors: electron,
muon and tau. In theory, heavy water should register the arrival of all
three flavors with equal probability,
regardless of how they try to hide by
oscillating from one flavor to another.
Building SNO took 15 years, and its
heavy water alone, lent for the experiment by Atomic Energy of Canada Ltd., is valued at $210 million.
There were many delaying snags
along the way, and yet it took only a
few days to begin seeing the first
neutrinos after the detector was inaugurated in April, say the Canadian, American and British scientists
who built it.
Dr. Arthur B. McDonald, the director of the observatory, said in his
announcement on June 9 that "to see
such clear examples of neutrino interactions within days of finally turning on was a real triumph for the
entire SNO team."
Dr. Eugene Beier of the University
of Pennsylvania, a spokesman for
the United States contingent of the
multinational observatory team,
said it would probably take about two
years before the detector yielded
"definitive" evidence of having spotted all three neutrino flavors.
Meanwhile, the detector may have
to be modified to improve its sensitivity. In one experiment, magnesium chloride would be mixed with
the heavy water, because chloride is
particularly effective in capturing
neutrons emitted by neutrino interactions. In another modification, detectors containing the rare isotope
helium-3 would be added to the water
tank; helium-3 offers neutrons an
even fatter target.
At stake is a hoped-for confirmation that neutrinos in flight oscillate
between flavors. Under the rules of
quantum mechanics, this implies
that neutrinos have mass, a situation
with enormous implications for astrophysics and cosmology because
neutrino mass could affect the rate
of expansion of the universe and influence the formation of galaxies and
large-scale structure in the universe.
Two new developments in Japan
are narrowing the search for confirmation of neutrino oscillation.
In a paper to be published next
week, a large Japanese and American team reports the discovery of a
nonsymmetric effect in the arrival of
neutrinos at the Super Kamiokande
detector. More neutrinos were found
hitting the detector from the western
direction than from the east, an effect predicted from Earth's magnetic field.
Neutrinos themselves are not influenced by magnetic fields, but in
this case they were created by the
annihilation of positively charged
cosmic ray particles drawn toward
Earth by the planet's geomagnetic
field. Neutrinos created by the impact of cosmic-ray particles on upper atmospheric atoms continue
along roughly the same trajectories
as the original cosmic rays.
The asymmetric east-west neutrino result came as no great surprise
to the experimenters, "but it was an
extremely important result," Dr.
Beier said, "because it showed by
independent means that the measurements of neutrino flow reported
in Japan last year were made correctly."
Another important neutrino experiment making use of a powerful
proton accelerator in Japan is expected to begin in a few days, said
Dr. Lawrence R. Sulak of Boston
University, a member of the Super
Kamiokande research team.
In the experiment, a proton beam
from Japan's KEK (a Japanese acronym meaning High Energy Accelerator Research Organization) is to
be fired into an aluminum target
producing an intense beam of pi-meson particles, which decay rapidly into neutrinos and muon particles. The resulting neutrino beam
will first pass through a nearby water detector and then hit the ground
at a downward angle of 1.5 degrees,
re-emerging 155 miles to the west at
the Super Kamiokande detector.
(The experiment is known as K2K,
for KEK to Kamiokande.)
The 155 miles of Earth through
which the beam passes offers no
appreciable barrier to the neutrinos.
If the same neutrino flux is found at
the nearby detector as at the distant
Kamiokande detector, the experiment will offer no proof of oscillation.
But if, as expected, fewer neutrinos
are found at the end of the line than
at the beginning, it will mean that
detectable neutrinos have transformed themselves into an undetectable form on their way through
Earth's crust.
The experiment is expected to take
about two years to yield solid results,
Dr. Sulak said.
Meanwhile, somewhat similar experiments are planned in Europe,
where a beam of neutrinos from the
Fermi National Accelerator Laboratory near Chicago will slice through
Earth to a neutrino detector 460
miles away in an iron mine near
Duluth, Minn.
In a third project, a neutrino beam
from the European Laboratory for
Particle Physics (CERN) near Geneva would be directed through the
ground to detectors within the Gran
Sasso Tunnel under the Italian Alps
455 miles away.
"Many experiments are closing in
on the neutrino from many different
angles," Dr. Sulak said. "With luck,
it won't be too long before we penetrate the particle's secrets."
Related Sites
These sites are not part of The New York Times on the Web, and The Times has no control over their content or availability.